by: Markus Deserno, Muhammed Ergüder, Samuel Foley, Amirali Hossein, and Malavika Varma
Living cells and many of their internal organelles are bounded by a membrane composed predominantly of lipids. These are molecules with a water-soluble head region and a water-insoluble tail region. As a result, they form a bilayer structure, with the tail regions facing each other and the head regions facing the water. Lipids come in a variety of types, and the lipid composition differs between the two sides of a biomembrane.
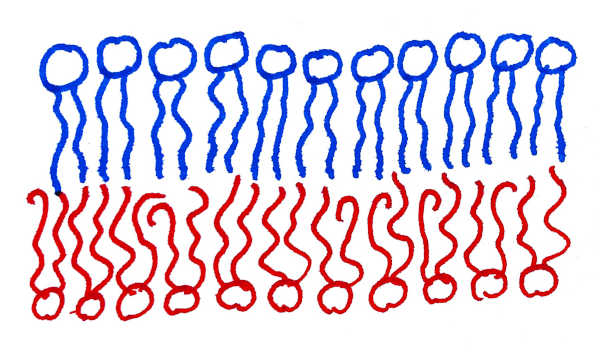
Fig 1: Asymmetric lipid membrane, with a more ordered lipid species in the upper leaflet, and a more disordered lipid species in the lower one.
While this asymmetry has been known and characterized to some extent since the 1970s, a recent paper published in Nature Chemical Biology by Lorent et al. takes this to a new level, offering detailed insight into the composition and asymmetry of cellular plasma membranes—the membranes surrounding cells.
A single type of lipid is sufficient to make a lipid membrane. Given that, it is puzzling that cells build their biomembranes from mixtures of hundreds of different types of lipids. It is not clear why they do this. Moreover, membranes artificially created in the laboratory contain the same composition of lipids on its two sides, but biomembranes are often distinctly asymmetric in that regard. Again, the reason for this is not known. This structural complexity of cellular membranes is one of the big mysteries of cell biology, and one of the first steps of solving it is to gain a better understanding of this complexity.
In the past, lipid molecules from membranes were identified using chromatography. This is similar to the following well-known experiment: place an ink drop at the bottom of a filter paper strip; if one lets water wick through the strip, the drop is spread into multiple different colors, thereby revealing its composition. An analogous technique was used to separate different lipid types, but this becomes increasingly difficult for a large number of similar molecules. In the case of plasma membranes, this number is in the hundreds. Lorent et al. instead use the much more sensitive technique of mass spectrometry, which differentiates lipids by accelerating them through electric and magnetic fields.
It has been known for several decades that the outer leaflet of the cell’s plasma membrane has a large number of lipids whose hydrocarbon tails do not contain any double bonds. Such lipids pack very well into ordered arrangements. In contrast, the inner leaflet of the plasma membrane hosts a much larger fraction of lipids which do have double bonds. These lipids tend to form much more disordered arrangements. Lorent et al. confirm this finding, but with the ability to distinguish lipid species with much finer granularity (e.g., tail lengths, number of double bonds, head types, etc.) than could previously be accomplished. This allows them to present a “lipidomic barcode”, which succinctly characterizes the precise composition of each leaflet of the membrane.
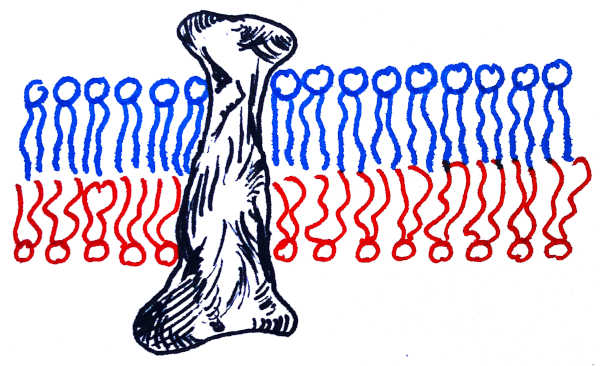
Fig. 2: Lipid membrane with an embedded protein. Notice that the part of the protein embedded in the upper ordered bilayer is thinner than the part embedded in the lower disordered bilayer.
In addition to lipids, biomembranes contain a myriad of proteins that either bind to the membrane surface (“peripheral”) or insert into it, passing through the bilayer from one side to the other (“transmembrane”). It was observed previously that the leaflet asymmetry of the plasma membrane is mirrored in the shape of the membrane-spanning portions of transmembrane proteins.
Lorent et al. go significantly beyond this finding: since the shape of that transmembrane region can be readily inferred from the protein sequence, one can infer the asymmetry of biomembranes belonging to intracellular organelles that are difficult or impossible to isolate and probe directly. For instance, their paper shows that the asymmetry of endosomal or lysosomal membranes very closely resembles that of the plasma membrane. In contrast, other organelles, such as the endoplasmic reticulum or the Golgi apparatus, have proteins with symmetric transmembrane regions, suggesting that their host membranes are symmetric as well.
Even more interestingly: Lorent et al. applied the same analysis to a wide variety of different organisms, such as amphibians, worms, and even fungi—a broad survey of “eukarya”, one of the three big domains of life. The plasma membranes in all these organisms were found to be asymmetric; in fact, they closely matched the asymmetry of our own cells. This leads to a deep conclusion: while we do not yet know the ultimate reason or purpose for biomembrane asymmetry, the fact that it is so widely conserved across such a broad spectrum of organisms implies that it must be fundamentally important.
This is an exciting result about the biological importance of membrane asymmetry, which underscores its ubiquity and poses a host of new questions. It also happens at a time where membrane biophysicists have devised several new experimental methods for artificially creating and examining asymmetric membranes. These provide a simplified test system for investigating the many ways in which asymmetric membranes differ from their symmetric counterparts, and how these differences could point us to the physiological purpose of asymmetry. For instance, it has been speculated that the higher degree of disorder in the inner leaflet of the plasma membrane makes it ideal for protein rearrangements and signaling, but at the same time the widely studied “lipid rafts”, believed to be signaling platforms, are formed in the outer leaflet. Uncovering how these connections work is important for understanding many human diseases that arise if such signaling goes wrong, which shows that exploring the physiological basis for asymmetry could help combat such diseases.
0 Comments