The basic structural component of cellular membranes are lipid molecules, of which a typical cell contains about a thousand different types. This is an amazingly large number, considering that a single species of lipid suffices to make a perfectly nice membrane. Over the past two decades biologists and biophysicists have come to appreciate that membranes are more than just fluid elastic sheets that compartmentalize cells and hold a host of embedded proteins in place. Rather, their fluidity, electric potential, phase behavior, lipid asymmetry, and other finer aspects play a crucial role for many of their functions. This, one might rightfully suspect, cannot all be had with one type of lipid, or a few. But why cells luxuriate in a lipidome that boasts such an incredible diversity remains one of the big mysteries of cell biology.
This diversity is furthermore astounding because it raises the question of control. Surely, if this elaborate lipid cocktail is functionally important, it cannot be random. Something needs to maintain the total lipid content and the relative fractions of lipid types comprising it at a well-defined set point. How is this done? Moreover, what precisely this point is depends on environmental conditions, for instance the temperature. This is known as the problem of lipid homeostatis, and in a recent paper in the Biophysical Journal, Martin Girard and Tristan Bereau propose an intriguing new biophysical perspective on it. Incidentally, the paper is accompanied by an excellent New and Notable by Thomas Shaw and Sarah Veatch, that is also very much worth your time!
Think about what needs to be explained. For each individual lipid type, the machinery making it needs to know how much it has to produce. This depends on how much is already there, what else is there, and what the present environmental conditions are. We need more than an army of controllable lipid manufacturing lines; we also need an army of sensors telling these lines how much of each lipid species to produce.
And if that were not enough, the re-tunings that have to happen are quite subtle: if for instance the temperature increases, certain lipid species tend to be upregulated, while others tend to be downregulated. Where are the instructions for that? What enormously complex array of sensors, response mechanisms, signaling networks, and feedback loops has nature set up to get cellular membranes “right”?
It is natural to approach this as a control problem, then ask “who does the controlling,” and then focus on the protein machinery that is involved. Girard and Bereau instead invite us to view this as a sensing problem. How would the cell know what to even change? Where are all these amazingly delicate sensors hidden that tell the cell what the current status is? The surprisingly elegant answer is: the membrane itself is the sensor.
Think about it: the cell apparently has a vested interest in a particular physical state of the membrane. It only cares about a specific lipid composition inasmuch as that composition realizes the physical property it cares about. Take the phase behavior and membrane order as an example. The cell wishes to maintain this at a given set point, but if the temperature increases, membranes become more disordered, and the cell needs to counterbalance that.
Girard and Bereau propose that a cell might not need to specifically adjust every component in a delicate lipid mixture such that lipid order stays put. Say, if it gets warmer, the cell could carefully ramp up the production of more saturated lipids and reduce the number of unsaturated ones, thus maintaining the degree of lipid order, but such an elaborate “hands on” approach might not be needed. Instead, cells could tune the concentration of a particular component that is known to be positively correlated with lipid order: cholesterol.
Why would that help? Why would the presence of one component even affect any of the others? Answer: because the collective thermodynamic state of the entire membrane determines how easy or hard it is to add or remove a given type of lipid.
As it turns out, and we have known this for a long time, cholesterol likes to be surrounded by ordered lipids. In more physical terms, the free energy of cholesterol is lower when it is surrounded by ordered lipids, and that in turn creates a higher incentive for ordered lipids to be added to a membrane that is rich in cholesterol. In fact, each lipid species has its own unique free energy of insertion into the membrane, called its “chemical potential”. The important thing to understand is that this chemical potential depend not only on the species in question, but also on the overall phase state of the membrane.
This is how the membrane becomes its own sensor: If two particular lipid species A and B differ in their chemical potential, the lipid insertion machineries will—for basic thermodynamic reasons—find it easier to embed the lipid with the lower chemical potential, say A. This creates a preference for inserting A, and so the overall concentration of A in the membrane increases. But there is a trade-off: as A becomes more abundant, it also becomes less favorable to keep pushing even more A into the membrane, largely for entropic reasons. The system hence self-stabilizes at a new concentration of A. Ultimately, if the chemical potentials of all lipids have reached the same value, it no longer matters which specific lipid gets inserted, and the system has reached a new stable equilibrium state. “Stable” in the sense that any deviation from it will re-activate the bias and “repair” that deviation.
Controlling the cholesterol concentration in a membrane can hence be viewed as a “master knob” for controlling its order. Turning it will induce many other changes in the lipidome. Girard and Bereau explicitly demonstrate this in simulations in which they permit lipids to “change their type”, i.e., change their number of double bonds and with it the degree of unsaturation (as a simple way to mimic the workings of an underlying lipid extraction and production machinery). Girard and Bereau show that tuning cholesterol levels leads to a reorganizing of the entire lipidome (which in this case only involves $16$ species—mirroring the number of ways $4$ distinct sites in the lipid tails could or could not hold a double bond: $16=2^4$). Hence, if the temperature goes up and membranes consequently become more disordered, there is only a single thing the cell needs to do: turn the cholesterol knob up by a judiciously chosen amount. The rest will happen automatically.
Of course, this is a simplified model case. Cells definitely want to control more than just membrane order. But the basic idea that the thermodynamic state of the membrane may act as a sensor for the very thing the cell wants to control, and that it might only be required to tune the concentrations of a few key drivers (“knobs”) to affect large-scale correlated adjustments of its entire lipidome, is probably right. Cells don’t have to keep track of the many intricate ways in which a complex lipid mixture leads to a complex physical state. Thermodynamics does most of this all by itself.
If so, this is also good news for biomembrane science: if the cell doesn’t actually have to bother understanding the intricate details of its lipid composition, maybe scientists don’t have to understand it either? Maybe it suffices to find what those few precious knobs are, and what physical property they end up controlling. Maybe that will help us to not be distracted by all those trees if we want to see the forest? Sorry, be distracted by all those lipids if we want to see the membrane?
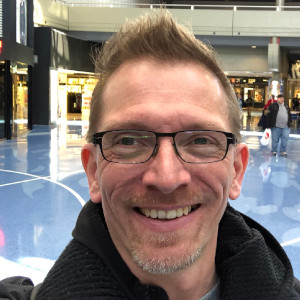
Markus Deserno is a professor in the Department of Physics at Carnegie Mellon University. His field of study is theoretical and computational biophysics, with a focus on lipid membranes.
0 Comments